Adhesiveness Performances of Monopetalanthus Durandii Tannin
Received Date: August 07, 2020 Accepted Date: August 31, 2020 Published Date: September 02, 2020
doi: 10.17303/jnsm.2020.6.101
Citation: Achille Gnassiri (2020) Adhesiveness Performances of Monopetalanthus Durandii Tannin. J Nanotech Smart Mater 3: 1-14.
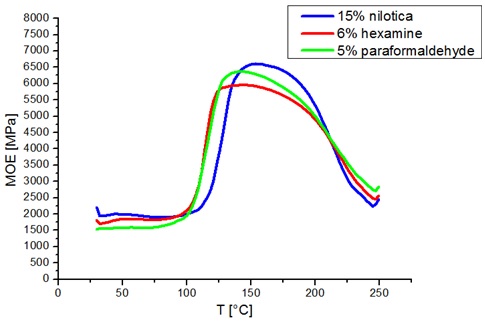
Abstract
The spectroscopic and spectrometric methods such as MALDI TOF and FT MIR, thermomechanical analyzer and the Stiasny number were used to determine the adhesive performance of Monopetalanthus durandii tannin. This extract is a condensed tannin with the repetition of 62-64 Da corresponding to Chrysin (256 Da) and dicationized (2xNa+) form. The MOE of the bio adhesive developed using bio hardener at natural pH and its Stiasny number are respectively 6608.65 MPa and 74.2%. A UD biocomposite has been developed based this bio adhesive had very good tensile properties. Its tensile strength (MOR), young’s modulus (MOE), yield strength (Rp0.2) and plastic deformation (ε0,2) are respectively 72 MPa; 8 GPa; 38.1 MPa and 0.7%.
Keywords:adhesiveness, spectroscopic and spectrometric methods, condensed tannin, UD biocomposite, tensile properties
Introduction
In the last decades, wood panels (particles, fibres, etc.) commonly used in the building sector have been bonded industrially with synthetics resins. These resins have several negative consequences (pollution, diseases) for the environment. The adhesives used in manufacturing these panels were mainly urea-formaldehyde or phenol-formaldehyde resins the formaldehyde having been classed as oncogenic [1], The U.S. National Toxicology Program, in its 12th Report on Carcinogens published in June 2011, has provided sufficient evidence of the carcinogenicity of formaldehyde. The National Agency for Food and Environmental Health Safety recommends preventive actions (substitution of formaldehyde, concentration limits, etc.) and the restriction of the uses of formaldehyde for certain applications (construction products, MDF, MDP, etc.) [2]. Thus, the wood industry is confronted with environmental problems which require alternatives solutions. These studies represent a growing field of research and many projects involving industry and universities have been carried out on this subject for several years [3–12]. Wood is a resource containing polymerizable components such as tannins, which have become of considerable interest for wood adhesives. Several tannins have been characterized in order to see their effectiveness in the development of wood adhesives. [4, 13–17].
Cameroon's flora represents 22,000 ha, i.e. 42% of the national area, with 300 available species, 80 of which are exploited by forestry companies, producing more than 1.2 million tons of residues each year [18]. Studies to enhance the tannin production of certain species of Cameroonian forests have been carried out for use as an adhesive in board manufacture [7, 15] and in pharmaceuticals [19–21]. Despite the potentially abundant supply in the tropical forests of the Gulf of Guinea, studies to detect and develop tannin production remains a vague area that deserves special attention.
The Monopetalanthus durandii also called Ekop mayo (Cameroon) is a generally cylindrical and fairly high tree (30 to 40 meters), present throughout West Africa, from Guinea to Zaire, but which is more abundant in southern Guinea. (Gabon, Cameroon, Congo). The reddish bark, smooth and 10 to 15 mm thick, is usually discarded in the wild by logging companies after wood processing. Like all other discarded materials, it is of interest to recycle them to avoid increased pollution.
In this paper, an ATR FT-MIR and MALDI ToF study of the tannin extract from the bark of Monopetalanthus durandii is carried out, and then used for the formulation of a 100% biosourced and high-performance composite resin with plant fiber reinforcement.
Materials and methods
Bark extraction
The bark was collected by a forestry company in the city of Douala called "Inter bois" located in the Bonamoussadi district. Douala is the economic capital of Cameroon, in the center of Central Africa, with a multitude of companies exploiting and transforming wood.
The bark pieces were dried at 29°C to a relative humidity of 30% and then crushed using hammer mills to obtain a powder with a grain size of 1-1.2 mm.
Tannin extraction
200 g of bark powder were mixed in an aqueous solution with a volume of water equal to 6 times the volume of bark, then placed in a water bath heated to 75°C and stirred for 3 hours. The suspension was then filtered using a Buchner funnel with a paper filter. The reddish-black liquid fraction obtained was concentrated by using a rotary evaporator at a temperature of 55°C and then dried at a constant temperature [14].
The tannin yield was calculated as follows (1):
yield(%)=((total weight of solids in the extract-total weight of sodium salts used))/(dry bark weight )
Extraction of Vachellia nilotica exudats biohardeners
A knife was used to scratch the bark of an Acacia nilotica tree in several places. The scratches were between 0.5 and 1 centimeter deep. After 30 minutes, the sap coming out was collected and then dried at room temperature (37°C) for 21 days. The dried exudates were finally crushed to obtain a whitish soluble powder easier to store and use. [7].
Tannin Characterization
ATR-FT MIR analysis
On the diamond/ZnSe crystal of the FTIR spectrophotometer PerkinElmer-Frontier of the University of Lorraine in France, were placed about 2 mg of fine powder of Monopetalanthus durandii tannin extract and then a manual force of about 150 N (hand force) was applied to the sample to obtain contact. Each spectrum is obtained by 32 scans and a resolution of 4cm-1 ranging from 4000 to 650cm-1 [4].
MALDI-Tof Analysis
The tannin powder extract was dissolved in an acetone solution (5 mg/ml) and then another acetone solution (10 mg/ ml) containing the matrix (2,5-dihydroxybenzoic acid) was prepared. To improve ion formation, NaCl was added to the matrix. The two previous solutions were mixed in a 50:50 ratio and about 0.5 to 1 µl of this mixture was placed on the sample holder. The sample holder was then introduced in the spectrometer (Aixima Performance, Shimadzu UK, Manchester, UK) after evaporation of the solvent for a few minutes in the open air [15].
Determination of tannin content (reactive tannin)
50ml of tannin solution (0.4% w/w) was pipetted into a 150 ml flask. Then 5 ml of a 37 % aqueous solution of formaldehyde and 5 ml of hydrochloric acid (10M) were added in turn and the mixture was heated under reflux for 30 min. At the end of this reaction, the hot mixture was filtered through a sintered glass filter (40-100 µm). The precipitate was dried at a constant weight in an oven at 105° C. The Stiasny number is the ratio of the oven-dried weight of the precipitate to the total dissolved solid content of the tannin extract, expressed as a percentage (2). [14] :
Stiasny number (%)=(Oven-dried weight of precipitate x100)/((Dissolved solid content/100)×50)
Resin formulation
A 40% tannin solution at natural pH was prepared by gradually adding the tannin powder under mechanical stirring to avoid the formation of tannin lumps in the resin. The hardener was then gradually added (by weight on the dry weight of tannin). The mixture was then kept under mechanical stirring for 8 minutes to ensure homogenization of the resin. The components of the different formulations F1, F2 and F3 are specified in Table 1
Resin characterization
Thermomechanical analysis
Triplicate samples of two beech-wood plys each 0.6 mm thick with 25-30 mg of the liquid resin placed between the two, for a total samples dimensions of 21 x 6 x 1.2 mm, were tested in non-isothermal mode between 40 and 220°C at a heating rate of 10°C/min with a Mettler Toledo TMA/SDTA840 TMA apparatus in three points bending on a span of 18 mm exercising a force cycle of 0.1/0.5N on the specimens with each force cycle of 12s (6 s/6 s), according to already established procedures [4, 22–24]. The maximum of the modulus of elasticity curve as a function of temperature represents the modulus of elasticity (MOE)
Viscosity
The viscosity of the samples was measured using a Brookfield RV viscometer (Brookfield, ES distributors, Garches, France) equipped with a number 5 spindle containing, operating at 20, 50, 100 rpm at 25°C.
Density of Resin
The density was determined in accordance with ISO 2811, using an elcometer 1800 pycnometer with a capacity of 50cm3 . Tests were carried out in a chamber at 20°C. The density is calculated using the formula (3):
density=((weight of the full pycnometer [g]– weight of the empty pycnometer [g]))/(Pycnometer volume [ml]) (3)
Manufacture of biocomposites
Flax fibres were obtained from the “Centre d’Essais Textile de l’Université de Lorraine” (CETELOR, Epinal, France), combed and then used for weaving unidirectional (UD) mats. The fibres have not been treated chemically before being used. The resulting mat had a thickness of 10mm, a surface area of 200x175mm2 and a mass per unit area of 2057g/m2 and was then conditioned in a 20°C chamber for 7 days before use.
The UD mat was homogeneously impregnated with a foulard (manufactured by Mathis, Zurich, Switzerland) and placed between two cylindrical rollers with resin between them. After impregnation the mats were dried to a moisture content of 20%.
The impregnated UD mat was thermo-pressed in a hydraulic press Gotfried Joos Maschinenfabrik (D-72285, Pfalzgrafenweiler, Germany) of the Laboratory-Press panel type, at a temperature of 150°C, according to a cycle as shown in Table 2.
Tensile Test of Biocomposites
The composites were cut according to the NF ISO 527- 4/2/1 standard, then stored for 3 days at a temperature of 20°C and a relative humidity level of 65%, so that the samples had the same humidity before testing. The tests were carried out on an INSTRON 4020 testing machine with a load cell of 30 kN, at a test speed of 1 mm/min and a breaking point of 90%. Seven samples were tested, test data was collected and stress-strain-curves were plotted using Origine Pro 8.5 software to determine tensile strength, Young’s modulus (MOE), yield strength (Rp0.2) and plastic deformation (ε0,2).
Results and discussion
Tannin Characterization
ATR-FT MIR analysis
The ATR-FT MIR spectra in Figure 1 show typical peaks of tannin extract in the 500 and 4000 cm-1 ranges. Each peak in this spectrum corresponds to a specific functional group. The wide, pointed band at 3187 cm-1 indicates the presence of O-H from flavonoids. The vibratory movement of the C=C group attached to the aromatic ring is confirmed by the presence of the 1602, 1507 cm-1 and 1112 cm-1 peak. These peak areas, which are fairly narrow and not very intense, are particularly indicative of the presence of condensed tannin. The presence of a C-O group attributed to the gallic/pyrogallic ring and the vibrations of the Gallocatechin results in the 1448 cm-1 band. The 1232 cm-1 band highlights the B-ring catechol-like vibrations occurring in the Fisetinidine and Catechins while the signals 1157, 1112 cm-1 represent the vibration of the resorcinol-like ring A of Robinetinidines and Fisétinidines [17]. The presence of the Ether group is shown by the presence of the 1112 cm-1 signal. This peak particularly reflects the stretching of the C-O bonds of the non-aromatic cyclic ring of polyflavonoids. [17].
Maldi-Tof Analysis
The spectra from the MALDI-ToF analysis of the tannin extract of Monopetalanthus durandii from the Figure 2 (a), b), c) et d)) shows monomers either without or with Na+ such as Catechin (290.2 Da), Gallocatechin gallate (458,3 Da), Chrysin (254.2 Da), Myricetin (318.2 Da, as its Na+ form at 340.8 Da), Fisetinidin (274.6 Da), Chalcon (208,2 Da), Gallocatechin (306.3 Da) and Catechin gallate (442,3 Da) between 50 and 1800 Da. The mass of the Na+ matrix used (23 Da) of the NaCl used was taken into considerations in the calculations, when this was present. It was subtracted from each peak to obtain the value of the molecular weights of the species present in the MALDI spectra [4].
The spectra show, apart definite flavonoids monomers and oligomers the existence of a repeating motive of 62 Da-64 Da, except for 1128.3 - 1160,3 Da and 1352,1– 1384,6 Da where the difference is 32 Da (64/2). As no repeating monomer units of a molecular weight 62-64 Da exists it becomes evident that there is flavonoid unit that is added to different other monomers or oligomers presenting a molecular weight equal to a multiple of 62-64 Da. The most likely flavonoid species fulfilling this role is chrysin, of molecular weight 256 Da when not linked and 255 Da or 254 Da when singly or doubly linked, respectively. This causes the formation of a series of oligomers in which chrysin is added to different flavonoids each of different molecular weight. This is true except for the 1128.3 - 1160,3 Da and 1352,1– 1384,6 Da peak ranges for which the difference is 32 Da. Moreover, the structure of chrysin is such that it can only be linked by C6 and C8 to other flavonoids in a chain. Thus, the oligomers so formed can be defined as an “angular tannins”. This means presenting the following aspect in which two other flavonoid units are linked to chrysine one C4-C8 and the other C4-C6 [14, 25–28].
The combinations of the different monomers of the peaks observed in the MALDI ToF curves in Figures. 2a-e are listed in Table 4. The oligomers except 312.1, 326.0, 340.6, 360.8, 374-418.6, 440.9-480.8, 495.7, 587.7, 600.4, 649.5, 805- 840.3, 867.2, 897.0, 959.1, 1021.0, 1083.1, 1112.2, 1160.3, 1207.0, 1352.1, 1641.0 Da, are protonated or multi-protonated. The protonated form is due to the presence of residual moisture in the test sample and once the components are protonated, even if they are dry, they are still protonated [4, 29]. The peaks 392,8 Da and 360,8Da have either gained a group (OH) and H2O respectively. This tannin extract is thus rather particular because of an accentuated presence of chrysin is observed as well as its dicationized (2 × Na+) forms justified by the presence of peaks localized at 364, 9 and 368 Da.
Stiasny number of tannin (tannin reactivity)
The tests were repeated six times and the average was used to calculate the Stiasny’s number (Table 5) that was 74.2%. This result shows that Monopetalanthus durandii is suitable for the application as an adhesive (at least 65%) as reported previously [30]. These numbers, although lower than those of Acacia mangium, can be explained because the Stiasny’s number varies according to the particle size of the bark during extraction (0.5–1 mm, Stiasny=82,1% ; 2–3 cm, Stiasny=79,9%) for Acacia mangium [31], A long extraction time could also significantly increase the Stiasny’s number (56,4% at 2h, 80,8% at 3h, 24,2% at 4h 48,8% at 5h) [31]. The Stiasny’s number is also influenced by the site where the plant grows, the method and the yield of extraction. Table 5 gives an example the Stiasny’s number different values according to the extraction sites with the relative yields. A comparison of the yield of water-extracted tannin with sodium-extracted tannin sulfite/carbonate showed that the latter yielded 30% more tannin [31]. Although tannin yields influence tannin reactivity, Lembah Beringin’s Acacia mangium has a yield of 89.1% for a yield of 11.2 higher than that produced at Telaga which has a high yield (20.3%) with the Stiasny number only 70.1. This could explain the difference between the values obtained in our study.
Resin characteristics
Gel time and Viscosity
The different resins after formulation were tested for viscosity (Table 3). The Gel times of each resin as a function of pH are given in Figure.4 in the pH range 6.3 - 10 and can be observed to increase exponentially with the pH (Figure. 4a) and decrease with the increase of pH (Figure. 4b). The gel time test evaluates the reactivity of the tannin with an aldehyde under the same conditions. The fastest gel time is at pH 10 for paraformaldehyde (48 s) and at pH 6.3 for hexamine (198 s) as hardeners. The gel time of the resin with formaldehyde is slower than for pine tannin (26 s)[32] and slightly faster than mangrove tannin (50 seconds) [33]. The tannin of Monopetalanthus durandii formulated with 6% hexamine as hardener, reacts very rapidly at natural pH, faster than mimosa tannin under the same conditions [9]. It is to note that the extraction process of the different tannins may have an impact on the reaction with the aldehydes and also the different solutions used. Hexamine does not decompose into formaldehyde in the presence of flavonoid tannins, but reacts by a different mechanism [9, 34], This is interesting because it does not produce any formaldehyde emissions when it is used [9, 35]. The gel time of the resin with the Vachelia ni lotica hardener gives relatively long gel times with the tannin of Monopetalanthus durandii. This could be explained by the fact that it does not polymerize at 100°C, as can be seen on the TMA curve in Figure. 5. The viscosity of the resin has an influence on the determination of the gel time, research has shown that the long gel times at the natural pH of resins based on biohardeners could be due to the evaporation time of the water contained in the resin [7, 36], higher viscosity, reduced gel time. At natural pH, Maritime pine tannin resin hardened with Vachellia nilotica (450 Cp ; 1500 s) [36] and G. dewevre tannin resin hardened with Vachellia nilotica (950 Cp ; 584 s) [7] differ most likely because the real hardener hidden in the exudate of V.nilotica is 2,5-dihydroxymethylfuran, which would not react like aldehydes [36]
Thermomechanical Analysis of Resins
The observation of ATM curves shows three phases: evaporation, solidification and degradation. F1 resin with 15% bio hardener (V.nilotica), has a higher modulus of elasticity than F2 and F3 resins. The TMA results indicate that the best performance is estimated by the highest value of the MOE obtained [37, 38]. F2 and F3 resins polymerize at practically the same temperature (100°C), faster than the bio-resin which polymerizes at 115°C, proving moreover that the 2,5-dihydroxymethylfuran in the exudates is slower-reacting than the aldehydes in synthetic hardeners.
Biocomposite processing and tensile testing
The biocomposite manufactured is a completely biobased material without the addition of NaOH in the resin. Its density is 978 g/m3 with a fiber/matrix ratio of 60/40. Figure 6b) and 6c) illustrate the good cohesion of the resin with the fibres, although there is a remarkable presence of pores on the surface of the composite due to the evaporation of water during thermoforming, we also observe in Figure.6(c) some fibers that are not in cohesive with the resin. These two observations could decrease the mechanical characteristics of the composite because it would decrease the charge transmission from the totality of the fibres to the matrix. To remedy this, a surface treatment must be applied to the fibres before weaving the mats.
The characteristic curves of the tensile tests, shown in Figure 7, reveal a similarity in the behaviour of the different specimens. with different phases typical of a ductile material. It results from this a tensile strength (MOR=72 MPa); a modulus of elasticity (MOE=8 GPa); an elastic strength (Rp0.2= 38.1 MPa) and a plastic deformation (ε0,2= 0.7%). It would be important to note that these properties could be improved by treating the fibres with a corona discharge to optimize the cohesion between the flax fibres and the resin that was observed on the test specimens.
Conclusion
The characterization of the tannin of Monopetalanthus durandii by MALDI-ToF, 13C NMR, ATR FT MIR indicates that it is composed of Catechin, Gallocatechin gallate, Chrysin, Myricetin, Fisetinidin, Chalcon, Gallocatechin and Catechin gallate. This tannin contains repetition of 62-64 Da corresponding to Chrysin (256 Da) and dicationized (2xNa+) forms for the peaks at 364,9 and 368 Da. This tannin was then used in the formulation of a resin to produce a biocomposite from a UD flax mat. The Stiasny number test indicated that this tannin could substitute phenol in a wood adhesive too. The resulting biocomposite had high-performance with good tensile characteristics. A study on the actual rate of hardening and the optimal pressing cycle of such biocomposites as well as the treatment of the fibres with corona before weaving the fibres mat would be an interesting approach to ameliorate the results.
- CIRC. (2006) IARC Monographs on the Evaluation of Carcinogenic Risks to Humans. Retrieved from https://www. cancer-environnement.fr/LinkClick.aspx?fileticket=pJAqhPPG_ RE%3d&tabid=302&portalid=0&mid=922
- Afsset. (2009) Cancers et environnement. Retrieved from www.cancer-environnement.fr › LinkClick
- Amziane, S., Collet, F., Lawrence, M., Picandet, V., Lanos, C., Marceau, S., Pavia, S. (2017) Bio-aggregate-based building materials. Aplication for hemp concrete, Ediia, 1: 1–11.
- Konai, N., Pizzi, A., Raidandi, D., Lagel, M. C., L’Hostis, C., Saidou, C., Ganash, A. (2015) Aningre (Aningeria spp.) tannin extract characterization and performance as an adhesive resin. Industrial Crops and Products 77: 225–231. https://doi. org/10.1016/j.indcrop.2015.08.053
- Motillon, C. (2013) Formulation et caractérisation de résines thermodurcissables bio-sourcées pour l’industrie du bois (PhD Thesis). Pau.
- Navarrete, P., Pizzi, A., Pasch, H., Rode, K., Delmotte, L. (2010) MALDI-TOF and 13C NMR characterization of maritime pine industrial tannin extract. Industrial crops and products 32:105–110.
- Ndiwe, B., Tibi, B., Danwe, R., Konai, N., Pizzi, A., Amirou, S. (2020) Reactivity, characterization and mechanical performance of particleboards bonded with tannin resins and bio hardeners from African trees. International Wood Products Journal 1–14.
- Ndiwe, B., Pizzi, A., Danwe, R., Tibi, B., Konai, N., Amirou, S. (2019). Particleboard bonded with bio-hardeners of tannin adhesives. European Journal of Wood and Wood Products 77: 1221–1223. https://doi.org/10.1007/s00107-019-01460- 5
- Pizzi, A., Kueny, R., Lecoanet, F., Massetau, B., Carpentier, D., Krebs, A., Ragoubi, M. (2009). High resin content natural matrix–natural fibre biocomposites. Industrial Crops and Products 30: 235–240. https://doi.org/10.1016/j.indcrop.2009.03.013
- Pizzi, A., et al. (1977) Hot-setting tannin-urea-formaldehyde exterior wood adhesives.
- Rhazi, N. (2015, December 29) Mise au point de mélanges collants écologiques à partir des écorces d’Acacia mollissima du Maroc (thesis). Pau. Retrieved from https://www.theses.fr/2015PAUU3049
- Saad, H. (2013) Développement de bio-composites à base de fibres végétales et de colles écologiques (PhD Thesis). Pau
- Haoua, K. (2010) Extraction et utilisation des Tanins de Marcs de Raisin pour la production d’adhésif pour le Bois (PhD Thesis). UHP-Université Henri Poincaré; INPL-Institut National Polytechnique de Lorraine.
- Hoong, Y. B., Pizzi, A., Tahir, P. M., Pasch, H. (2010) Characterization of Acacia mangium polyflavonoid tannins by MALDI-TOF mass spectrometry and CP-MAS 13C NMR. European Polymer Journal, 46: 1268–1277.
- Konai, N., Raidandi, D., Pizzi, A., Meva’a, L. (2017) Characterization of Ficus sycomorus tannin using ATR-FT MIR, MALDI-TOF MS and 13C NMR methods. European Journal of Wood and Wood Products, 75: 807–815. https://doi.org/10.1007/ s00107-017-1177-8
- Ramirez-Coronel, M.-A. (2004) Les tanins condensés de la pulpe de café: études structurales et dégradation enzymatique (PhD Thesis). Aix-Marseille 1.
- Tondi, G., & Petutschnigg, A. (2015) Middle infrared (ATR FT-MIR) characterization of industrial tannin extracts. Industrial Crops and Products 65: 422–428. https://doi. org/10.1016/j.indcrop.2014.11.005
- Saha Tchinda, J.-B. (2015) Caractérisation et valorisation des substances extractibles de cinq essences camerounaises majeures de l’industrie du bois: Ayous, Moabi, Movingui, Padouk et Tali (PhD Thesis). Université de Lorraine.
- Vandi, D., Nga, E. N., Betti, J. L., Loe, G. M. E., Ottou, P. B. M., Priso, R. J., Mpondo, E. M (2016) Contribution des populations des villes de Yaoundé et Douala à la connaissance des plantes à tanins et à anthocyanes. Journal of Animal &Plant Sciences 30: 4797–4814.
- Etchike, C. A., Sassa, A. M., Abba, A., Nyonbourg, E. (2011) Evaluation in vitro de l’activité antibactérienne de cinq plantes de la pharmacopée traditionnelle de l’Adamaoua (Cameroun). Cameroon Journal of Experimental Biology 7: 22–27.
- Lal, D., Shrivastava, D., Verma, H., & Gardner, J. J. (2012) Production of Tannin Acyl Hydrolase (EC 3.1. 1.20) from Aspergillus niger isolated from bark of Acacia nilotica. Journal of Microbiology and Biotechnology Research 2: 566–572.
- Garcia, R., & Pizzi, A. (1998) Crosslinked and entanglement networks in thermomechanical analysis of polycondensation resins. Journal of applied polymer science 70: 1111–1119.
- Pizzi, A. (1997) On the correlation of some theoretical and experimental parameters in polycondensation cross-linked networks. Journal of applied polymer science 63: 603–617.
- Pizzi, A., Probst, F., & Deglise, X. (1997) Molecular mechanics modelling of interfacial energy and flexibility on cellulose. Journal of adhesion science and technology 11: 573–589.
- Botha, J. J., Viviers, P. M., Young, D. A., du Preez, I. C., Ferreira, D., Roux, D. G., Hull, W. E. (1982) Synthesis of condensed tannins. Part 5. The first angular [4, 6: 4, 8]-triflavanoids and their natural counterparts. Journal of the Chemical Society, Perkin Transactions 1: 527–533.
- Pizzi. (1983) Tannin based adhesives in Wood Adhesives Chemistry and Technology ((A.Pizzi Ed),.). New York: Marcel Dekker.
- Roux, D., & Ferreira, D. (1982) The direct biomimetic synthesis, structure and absolute configuration of angular and linear condensed tannins. In Fortschritte der Chemie organischer Naturstoffe/Progress in the Chemistry of Organic Natural Products, Springer pp. 47–76.
- Viviers, P. M., Botha, J. J., Ferreira, D., Roux, D. G., Saayman, H. M. (1983). Synthesis of condensed tannins. Part 7. Angular [4, 6: 4, 8]-prorobinetinidin triflavanoids from black wattle (‘Mimosa’) bark extract. Journal of the Chemical Society, Perkin Transactions 1: 17–22.
- Saad, H., Charrier-El Bouhtoury, F., Pizzi, A., Rode, K., Charrier, B., Ayed, N. (2012). Characterization of pomegranate peels tannin extractives. Industrial Crops and Products 40; 239– 246. https://doi.org/10.1016/j.indcrop.2012.02.038
- Yazaki, Y., Collins, P. (1994) Wood adhesives based on tannin extracts from barks of some pine and spruce species. Holz als Roh-und Werkstoff, 52: 307.
- Hoong, Y. B., Paridah, M., Luqman, C., Koh, M., Loh, Y. (2009) Fortification of sulfited tannin from the bark of Acacia mangium with phenol–formaldehyde for use as plywood adhesive. Industrial Crops and Products, 30: 416–421.
- Pizzi, A., Cameron, F. (1994) Advanced Wood Adhesives Technology. Marcel Dekker. Inc., New York, 243–271.
- Paridah, M. T., Musgrave, O. (2006) Alkaline treatment of sulfited tannin-based adhesive from mangrove to increase bond integrity of beech slips. Journal of Tropical Forest Science 137–143.
- Kamoun, C., Pizzi, A., et al. (2000) Mechanism of hexamine as a non-aldehyde polycondensation resins hardener. Part 1: Hexamine decomposition and reactive intermediates. Holzforschung und Holzverwertung, 52: 16–19.
- Pichelin, F., Nakatani, M., Pizzi, A., Wieland, S., et al. (2006) Structural beams from thick wood panels bonded industrially with formaldehyde-free tannin adhesives. Forest products journal 56: 31.
- Ndiwe, B., Pizzi, A., Tibi, B., Danwe, R., Konai, N., Amirou, S. (2019) African tree bark exudate extracts as biohardeners of fully biosourced thermoset tannin adhesives for wood panels. Industrial Crops and Products, 132, 253–268. https://doi. org/10.1016/j.indcrop.2019.02.023
- Kamoun, C., Pizzi, A., Garcia, R. (1998) The effect of humidity on crosslinked and entanglement networking of formaidehyde-based wood adhesives. Holz als Roh-und Werkstoff, 56: 235.
- Lecourt, M., Pizzi, A., Humphrey, P. (2003) Comparison of TMA and ABES as forecasting systems of wood bonding effectiveness. Holz als Roh-und Werkstoff, 61: 75–76.
Figures at a glance