High-Resolution Ultrasound Changes of Vagus Nerve in Diabetes Neuropathy: A Possible Marker of Autonomic Parasympathetic Neuropathy
Received Date:September 03, 2020 Accepted Date:September 25, 2020 Published Date: September 30, 2020
doi: 10.17303/jnnd.2020.8.102
Citation:Sartucci F (2020) High-Resolution Ultrasound Changes of Vagus Nerve in Diabetes Neuropathy: A Possible Marker of Autonomic Parasympathetic Neuropathy. J Neurophysiol Neurol Disord 8: 1-9.
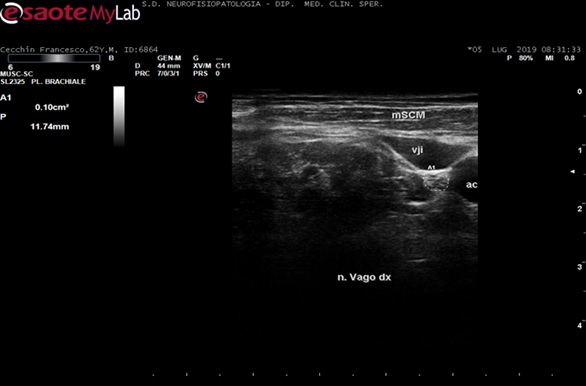
Abstract
Background and Rationale: somatic diabetic demyelinating distal sensory-motor polyneuropathy (D-DSP) represents a very common complication in diabetes mellitus (DM); autonomic neuropathy may be part of the disease and leads to high morbidity and mortality. Vagus Nerve (VN) is the longest autonomic nerve and commonly parasympathetic involvement precedes sympathetic symptoms. The aims of the present study were to assess sonographic changes of the VN in DM patients in cases with or without autonomic symptoms and neuropathy
Methods: The VN was scanned bilaterally in 20 healthy volunteers and 61patients (32 m, 29 f) with DM in the axial plane at the lateral neck. The acquisition of ultrasound images was performed using a 19MHz probe with an Esaote MyLab Gamma device; cross-sectional area (CSA), perimeter, echogenicity, and echo-structure were considered.
Results: Mean cross-sectional area of the vagus nerve was significantly smaller (3.13mm2 ; SD= 1.54) in patients with diabetes compared with controls (5.8 mm2 , DS = 1.2) and did not correlate with disease duration and parameters of cardiovagal functions.
Conclusions: our data prove a degree of vagus nerve atrophy in DM patients. This finding may have relevance in the diagnosis and management of diabetic neuropathy, overally on parasympathetic involvement, even if further investigations are needed to confirm the relevance of the findings.
Keywords:vagus nerve; diabetes mellitus; neuromuscular high-resolution ultrasounds; autonomic neuropathy; parasympathetic autonomic neuropathy.
Introduction
Diabetic demyelinating distal sensory-motor polyneuropathy (D-DSP) represents a common complication of Diabetes Mellitus (DM) [33, 36, 40] and several studies stated clinical and electro diagnostic criteria for diagnosing and estimating its severity [9, 24]. Inside D-DSP Autonomic Neuropathy (AN) represent a very severe complication of the disease. It involves multiple body apparatus and is associated with high morbidity and mortality [32, 35]. It commonly involves sudomotor, cardiovascular, gastrointestinal, and genitourinary systems [46]. Furthermore, vagal parasympathetic symptoms usually precede sympathetic ones [48].
The Vagus Nerve (VN), responsible of approximately 75% of the parasympathetic activity in humans, is the longest nerve of the autonomic nervous system [19] and can be damaged in the early phases of cardiac autonomic neuropathy CAN [11] represents a life-threatening complication and can be subclinical for several years [34]. VN is usually affected in D-DSP and neuronal degenerative processes have been previously rarely investigated and described in DM [18, 8] moreover, the exact pathogenic mechanism and meaning of morphological changes so far remain understood and debated [44].
In the last twenty years the utility of high-resolution ultrasound (HRUS) in the evaluation of peripheral nerves and their disorders has been widely proved and validated in many extensive studies [29, 51, 39, 14], including D-DSN [26, 31,1, 37]. Ultrasound represents a fast, easy, and noninvasive tool for the diagnosis of D-DSN [26, 37]. As far as concerns DM, enlargement of peripheral nerves has been generally reported [27, 21,3].
More recently, HRUS was employed to assess cranial nerve [13, 41,42,44]. Ultrasounds of VN for the assessment of DM neuropathy were rarely employed [4,15-17, 6, 43] and only a few data are available as far concerns vagal DM neuropathy [44]. Anywhere, due to the degree of resolution of HRUS with the modern device and transducer, HRUS could represent a powerful instrument, complementary to other investigations, and a formidable challenge for clinicians to detect VN involvement and pathological changes underlying its dysfunctions even at early stages of disease in DM.
The aim of this study has been to assess changes that may occur in VN in DM patients, either with or without D-SPN and autonomic manifestations. We based our rationale on the hypothesis that morphological changes of VN linked to the degeneration process in DM have been previously described in autopsy studies [8,18] and moreover could be in some extent visualized and estimated with HRUS.
Methods
Participants
Patients with DM were recruited during the 2016-2019 period from either outpatient and those of Section Department (SD) Endocrinology and Metabolism of Organ and Cell Transplants of the Department of Clinical and Experimental Medicine, Pisa University Surgery and Medical School. Control data for the VN cross-sectional area, perimeter, echogenicity, and structure, were obtained from volunteers, recruited among the staff of the Department, students, and specializing doctors in medicine and surgery
We excluded cases with other neuropathy and degenerative medical conditions that could mimic diabetic autonomic neuropathy. The age, sex, weight, and height of subjects enrolled were recorded, together with their body mass index (BMI). The VN was bilaterally scanned in 20 healthy volunteers (10 m and 10 f; mean age 65.2 + 10.3 yrs) and on the left side in 61patients with DM (32 m, 29 f; mean age 53.7 + 13.1 yrs) in the axial plane at the lateral neck. The demographic data of the two groups and the main clinical features of the patients are summarized in Table 1. There were no significant differences in age, sex, race, weight, and height, or BMI between the two groups.
A full medical history, encompassing onset, course, duration, and type of diabetes, was taken from every patient by one of the authors (EG). Specific attention was devoted to detecting autonomic symptoms/dysfunction to prove concomitant diabetic autonomic neuropathy. Symptoms of polyneuropathy were defined as reported numbness, tingling, or burning pain in the feet and hands, weakness, or muscle atrophy. The investigation assesses several domains, including orthostatic hypotension, sudomotor, vasomotor, gastrointestinal, urinary, and sexual dysfunctions.
Baseline clinical and laboratory measurements
All patients prior to being enrolled, after a detailed medical history, underwent a complete neurological and physical examination. Demographic data, including age, disease duration (years), body mass index (BMI), systolic blood pressure (SBP) and diastolic (DBP), micro vascular complications, and laboratory parameters were obtained and recorded at inclusion time. Of these, 46 were affected by a DMT I and 15by DMT II; mean disease duration was 27.26 yrs (SD = 11.68), BMI 25.06 SD = 5.89).
Assessment and scoring of nerve conduction investigations
Both presences of neurological symptoms/signs and abnormalities in nerve conduction velocity (NCS) and electromyography (EMG) studies were employed to diagnose and confirm D-DSP following the American Academy of Neurology [12,10]. All patients underwent standard nerve conduction studies (NCS) for polyneuropathy. Tests were performed using a Neuro travel EP/ERP Cadwell Sierra Wave machine (Ates Medica Device, Milano, Italy). For each patient, we investigated left sural sensory nerve conduction velocity (SNCV) and tibial motor nerve conduction velocity (MNCV), tibial F wave, and soleus H reflex [2, 22].
Among DM patients SNAP of sural nerve were absent in 32 patients (52.4 %); SNCV was reduced with or without a loss of SNAP amplitude in 29 (47.6%), Tibial nerve MNCV was not valuable in 5 (8.2%) patients due to absent Compound Muscle Action Potentials (CMAP) from abductor halluces brevis muscle; altered in 55 cases (90.2 %), within normal limits in 1 (1.6%). F waves mean latencies and dispersion were abnormal in all cases (absent in 16; 26.2 %). H reflex was abnormal in amplitude (H/CMAP amplitude ratio of less than 30%) and/or latency in all (100%). Needle EMG, performed bilaterally in tibialis anterior muscle and extensor hallucis longus, displayed neurogenic changes with loss of motor unit and spontaneous activity (membrane instability), more evident distally in all cases (100%).
On another day, all patients underwent tibial and sural HRUS: all cases exhibited some loss of CSA area measured at the ankle (sural = 2.36 mm2; Tibial = 3.5 mm2 ; v.n. > 5.1 and 8, 5 respectively for our laboratory).
Assessment and scoring autonomic functions
We performed in all cases Sympathetic Skin Responses (SSR) from left upper and lower extremities and in a small sample Valsalva heart rate variability (Valsalva ratio-VR) as described below [2, 28] following the Guidelines of the International Federation of Clinical Neurophysiology [7]. For these test, the same Neuro travel EP/ERP machine used for electro diagnostic investigations was employed.
SSR were absent in 7 (11.5%), abnormal in 46 (75.4%), within normal limits in 8 (13.1%). Valsalva Ratio, tested in 15 patients, was abnormal in 11 (73.4%), within normal limit in 4 (26.6%).
Vagus nerve imaging
A single, study-blind sonographer (FS) performed all US studies on a separate day from that of electrophysiological testing. The acquisition of ultrasound images was performed using a 19MHz linear array transducer using an Esaote MyLab Gamma device (Esaote, Genova, Italy); cross-sectional area (CSA), perimeter, echogenicity, and fascicular structure were considered and measured on the left side. The patients and controls were scanned by the same author (FS) at the Neuro physiopathology Section of the Department of Clinical and Experimental Medicine. The sonographer was blind to the autonomic involvement of patients or the results of electrophysiological testing.
Participants were scanned lying in the supine position while turning his/her head to the side opposite those investigated. The nerve was scanned in the axial view. Depth was set at 4 cm and the probe was placed first at the level of the thyroid lobe with the probe orientation marker directed toward the patient‘s right side. The probe was then moved laterally to identify the nerve inside the carotid sheath. Both the carotid artery and internal jugular vein served as anatomical landmarks. The VN was identified as a small rounded hypoechoic/or honeycomb structure widget deep to the Carotid artery and Jugular vein (see figure 1 for an example in a control subject). We measured the cross-sectional area of the VN by following the contour of the nerve just inside the hyperechoic rim with the probe exactly orthogonal to the nerve and with the least pressure applied; in addition, we considered the perimeter, echogenicity, and structure of the VN trunk.
Statistical analysis
Statistical analysis was performed using Sigma Plot version n. 12.0 package (Systat Software Inc, 2011-2012). Data were tabulated, calculated, analyzed; mean and SD of VN CSA area, and perimeter were determined. The measure obtained from the left side in controls and patients was used in the correlation and comparison studies. The patients were subdivided in groups according to the type of disease (DMT I or II) and the concomitant presence of autonomic dysfunctions.
Two-tailed Student’s test or Mann-Whitney Rank Sum Test were used to compare sonographic measures between controls and patients, side to side measures, and subgroups of patients’ findings.
Pearson correlation coefficient (Pearson Product Moment Correlation) was employed to correlate VN ultrasound measurements with age, weight, height, BMI, disease duration.
Results
The VN was easily visualized in both patients and controls between the carotid artery and the internal jugular vein. The mean cross-sectional area in our controls group was 5.8+1.2 mm2 ; in the DM group 3.13+1.54. Figure 2 shows an axial view of the VN in a DM patient. The range and mean value of VN CSA in the controls and patients groups are reported in Table 2. By comparing patients with controls, the mean CSA of the left VN was significantly smaller in patients compared to controls (P = < 0.001); side to side comparison of VN CSA in a sample (10 pts) revealed not significant inter side differences.
Mean VN CSA was 3.13 mm2 in patients with both DMTI and DMTII with no statistically significant difference between the two groups. Perimeter was 6.29 mm in DM group, 8.83 in controls (P = < 0.001).
Nerve trunk echogenicity was increased (hyperechoic) in 34 (55.7%), reduced (hypoechoic) in 24 (39.3%), isoechoic in 3 (4.9%) patients; a hyperechoic rim was detectable in 8 cases among the hyperechoic nerves (10.5%), in 3 (5.3%) among the hypoechoic ones. Nerve fascicular structure was detectable in 7 hyperechoic (11.5%); in 24 hypoechoic (39.3%), in 3 isoechoic (4.9%) nervous trunks. No one correlations with cardiovagal functions (Valsalva R-R variability) and sympathetic cholinergic activity (SSR) was evident (P> 0.005).
Also, CSA did not significantly differ between patients with autonomic symptoms/signs compared with that without. No significant correlation was detected among the VN CSA and age, disease duration, or BMI, both in controls and in patients. The same for the diabetic duration (P > 0.005 for all these parameters).
Discussion and Conclusions
Our values in controls are similar to the reference ones reported by other authors [5, 44] who scanned the VN either in controls as well as in DM patients. Our study confirms that VN CSA is significantly smaller in patients with DM compared to controls, providing an evident degree of VN atrophy even in absence of autonomic dysfunction. A cutoff value of 3.5 mm2 may be considered the right limit to define abnormal changes following data collected in the present study.
The VN degeneration has been previously described in patients with DM [8, 18, 44]. Guo and colleagues investigated pathological changes in VN removed at necroscopy in 3 pt with DM and found the low density of the myelinated fibers compared with controls; additionally, they found axonal degeneration. All these findings together can account US changes compatible with atrophy we detected in our group.
Our data seem conflicting with other previous studies that have demonstrated peripheral nerve enlargement in patients with definite diabetic peripheral neuropathy [47, 27, 31, 21, 3].
Traditionally it is believed that poor glycemic control represents a major risk factor for the development of D-DSP. Hyperglycemia leads to osmotic diffuse swelling of the nerve with secondary injury to the axons and myelin sheath, which triggers the onset of neuropathy [23]. The pathology of D-DSP is characterized by the progressive loss of myelinated nerve fiber and axons. The nerve swelling is thought to be the result of a cascade of several events: thickening of the vasculature, edema, proliferation of the basement membranes with perineural and Schwann cells; accumulation of microfibrillar material in the vicinity of perineural cells, and increased diameter of endoneurial collagen fibrils [50, 49].
However visibly smaller in size nerves have been reported in some root ganglion disorders [25] and distal axonopathy from taxenes [38]. In addition, it may be that neuropathy progressing to end-stage denervation, with scarring but without edema or a growth cone at a particular nerve site, might cause a relative decline in the cross sectional area [45]. In fact, we found atrophy in the sural and tibial nerve in our patient group who showed evident electrophysiological signs of denervation, loss of CMAP and SNAP amplitude providing evidence of a myelino-axonal loss; something that has been described in end-stage carpal tunnel syndrome [30]. Moreover, other previous studies in the field disagree [47, 27] and normal value has detected also in D-DSP [20]. It may be concluded that cranial neuropathy might not have so different pathogenesis compared with peripheral neuropathy in DM taking into account the earlier or later moment of the sonographic evaluation.
No significant differences were found in the VN CSA between patients with autonomic symptoms and those without; therefore vagal atrophy so far remains of uncertain functional significance in DM [44].
We noticed that the VN fascicular pattern also differed compared to controls, providing evidence of different patterns of VN involvement, whose meaning remains to be clarified. In addition, the BMI of controls was a bit not significantly lower than those of controls; moreover, results probably are not affected by BMI (p = 0.901, n.s.; Mann-Whitney Rank Sum Test).
Our study has some limitations; first, the relatively small number of patients enrolled, particularly those with severe parasympathetic involvement and future studies should include more of this type of cases. Another limit is the lack of a confirmation follow-up in the course of the disease.
In conclusion, the present study confirms VN atrophy displayable with HRUS that may represent an in-vivo replacement for simple histology; in addition prove to echo structural changes in patients with DM, give data useful for future investigations and application in clinical practice for early detection of VN involvement and above all precocious treatment of parasympathetic disorders in DM patients. Equally relevant investigate the evolution of these changes over time in autonomic neuropathy
US of the VN is a fast real-time powerful tool for detecting VN morphological changes in CAN, complementary to other techniques. Further studies with larger sample sizes will be necessary to confirm these results in DM and successful translation of these ultrasound approaches to the clinic could guide interventions to improve functional abilities in DM patients with benefit for either patients, their families, caregivers, and the scientific community.
Acknowledgment
We are grateful to Mattia Tomassini for his technical help and assistance in recording patients with DM.
Funding: research in the Clinical Neuro physiopathology Unit laboratory was supported by the research funds of the Pisa University Surgery and Medical School.
Author Contributions
FS: wrote and edited the manuscript, executed HRUS and performed a literature review of related topics, supervised the study; MS and TB: contributed in data collection, storing and analysis of data, as well as direct patient care; EG: analyzed clinical and processed statistical data; PM: provided patients and critically revised the manuscript for important intellectual content.
All co-authors have been substantially involved in the study and preparation of the manuscript; all read and approved the final version of the manuscript and accept the responsibility for its content
Conflicts of interest & Declarations
The authors declare that they have a noncompeting interest. The study involving human participants was reviewed and approved by the local Azienda Ospedaliera Universitaria Pisana Institutional Ethics Board.
The patients provided their written informed consent to participate in this study
- Arumugam T, Razali SN, Vethakkan SR, Rozalli FI, Shahrizaila N (2016) Relationship between ultrasonographic nerve morphology and severity of diabetic sensorimotor polyneuropathy. Eur J Neurol, 23: 354-360.
- Baron R and Ewing DJ: Heart rate variability (1999) In: Recommendations for the Practice of Clinical Neurophysiology: Guidelines of the International Federation of Clinical Neurophysiology (EEG Suppl. 52). Editors Deuschl and Eisen A. Elsevier Science B.V. 283-286.
- Breiner A, Qrimli M, Ebadi H, et al. (2017) Peripheral nerve high-resolution ultrasound in diabetes. Muscle Nerve 55: 171-178.
- Cartwright MS, Brown ME, Eulitt P, et al. (2009) Diagnostic nerve ultrasound in Charcot-Marie-Tooth disease type 1B. Muscle Nerve 40: 98-102.
- Cartwright MS, Passmore LV, Yoon JS, et al. (2008) Cross-sectional area reference values for nerve ultrasonography. Muscle Nerve 37: 566-571.
- Decard BF, Fladt J, Axer H, et al. (2015) Nerve ultrasound in Miller Fisher variant of Guillain-Barré syndrome. Muscle Nerve 52: 1106-1110.
- Deuschl G and Eisen A (1999) Recommendations for the Practice of Clinical Neurophysiology: Guidelines of the International Federation of Clinical Neurophysiology (EEG Suppl. 52). Elsevier Science B.V
- Duchen LW, Anjorin A, Watkins PJ, et al. (1980) Pathology of autonomic neuropathy in diabetes mellitus. Ann Intern Med 92: 301-303.
- Dyck PJ, Albers JW, Andersen H, et al. (2011) Diabetic polyneuropathies: update on research definition, diagnostic criteria and estimation severity. Diabetes Metab Res Rev, 27: 620- 628.
- England JD, Gronseth GS, Franklin G, et al. (2005) Distal symmetric polyneuropathy: a definition for clinical research. Report of the American Academy of Neurology, the American Association of Electrodiagnostic Medicine, and the American Academy of Physical Medicine and Rehabilitation. Neurology 64: 199-207.
- Ewing DJ, Martyn CN, Young RJ, Clarke BF (1985) The value of cardiovascular autonomic function tests: 10 years experience in diabetes. Diabetes Care 8: 491-498.
- Feldman EL, Stevens MJ, Thomas PK, Brown MB, Canal N, Green DA (1994) A practical two-step quantitative clinical and electrophysiological assessment for the diagnosis and staging of diabetic neuropathy. Diabetes Care 17: 1281-1289.
- Giovagnorio F, Martinoli C (2001) Sonography of the cervical vagus nerve: normal appearance and abnormal findings. AJR Am J Roentgenol 176: 745-749.
- Gonzalez NL and Hobson,-Webb LD (2019) Neuromuscular ultrasound in clinical practice: a review. Clin. Neurophysiol Pract. 4: 148-163.
- Grimm A, Decard BF, Bischof A, et al. (2014) Ultrasound of the peripheral nerves in systemic vasculitic neuropathies. J Neurol Sci 347: 44-49.
- Grimm A, Decard BF, Schramm A, et al. (2016) Ultrasound and electrophysiologic findings in patients with GuillainBarre syndrome at disease onset and over a period of six months. Clin Neurophysiol 127: 1657-1663.
- Grimm A, Thomaser AL, Peters N, et al. (2015) Neurological picture. Vagal hypertrophy in immune-mediated neuropathy visualised with high-resolution ultrasound (HR-US). J Neurol Neurosurg Psychiatry 86:1277-1278.
- Guo YP, McLeod JG, Baverstock J (1987) Pathological changes in the vagus nerve in diabetes and chronic alcoholism. J Neurol Neurosurg Psychiatry 50: 1449-1453.
- Guyton AC, Hall JE (2006) The autonomic nervous system and the adrenal medulla. In: Textbook Of Medical Physiology. 11th ed. Philadelphia: Elsevier 750.
- Hobson-Webb L, Massey MJ, Juel VC (2013) Nerve ultrasound in diabetic polyneuropathy: correlation with clinical characteristic and electrodiagnostic testing. Muscle and Nerve 47: 379-384.
- Kelle B, Evran M, Balli T, et al. (2016) Diabetic peripheral neuropathy: correlation between nerve cross-sectional area on ultrasound and clinical feature. J. Back Musculoskelet Rehabil 29: 717-722.
- Kimura J (2014) Electrodiagnosis in Diseases of Nerve and Muscle. Principles and Practice Oxford University Press Inc.
- Kunwar PS, Kamlesh G, Nilanshu K, Vijinder A (2020) High-resolution ultrasonography of the sural nerve in diabetic peripheral neuropathy. J Ultrason : e83-e89.
- Lai RY, Huang CC, Chiu WC, et al. (2019) Close relationship between cardiovagal function and sural sensory nerve action potential in type 2 diabetes. Clin Neurophysiol 130: 1160- 1165.
- Leadbetter R, Weatherall M, Pelosi L (2019) Nerve ultrasound as a diagnostic tool for sensory neuropathy in spinocerebellar ataxia syndrome. Clin Neurophysiol 130: 568-572.
- Lee D, Dauphinee DM (2005) Morphological and functional changes in the diabetic peripheral nerve: using diagnostic ultrasound and neurosensory testing to select a candidate for nerve decompression. I Am Podiatr Med Assoc 95: 433-437.
- Liu F, Zhu J, Wei M, et al. (2012) Preliminary evaluation of the sural nerve using 22-MHz ultrasound: a new approach for evaluation of diabetic cutaneous neuropathy. PLoS One 7: e32730.
- Low PA (2003) Testing the autonomic nervous system. Semin Neurol, 23: 407-421.
- Martinoli C, Schenone A, Bianchi S, et al. (2002) Sonography of the median nerve in Charcot-Marie-Tooth disease. AJR Am J Roentgenol 178: 1553-1556.
- Moghtaderi A, Sanei Sistani S, Sadoughi N, HamedAzimi H (2012) Ultrasound evaluation of patients with moderate and severe carpal tunnel syndrome. Prague Med Rep 113: 23-32.
- Moon HI, Kwon HK, Kim L, Lee HJ, Lee HJ (2014) Ultrasonography of palm to elbow segment of the median nerve in different degrees of diabetic polyneuropathy. Clin Neurophysiol, 125: 844-848.
- O’Brien IA, McFadden JP, Corrall RJ (1991) The influence of autonomic neuropathy on mortality in insulin-dependent diabetes. Q J Med 79: 495-502.
- Partanen J, Niskanen L, Lehtinen J, Mervaala E, et al. (1995) Natural history of peripheral neuropathy in patients with non-insulin dependent diabetes mellitus. N Eng J Med, 333: 89- 94.
- Pop-Busui R (2010) Cardiac autonomic neuropathy in diabetes: a clinical perspective. Diabetes Care 33: 434-441.
- Rathmann W, Ziegler D, Jahnke M, et al. (1993) Mortality in diabetic patients with cardiovascular autonomic neuropathy. Diabet Med 10: 820-824.
- Sharma KR, Cross J, Farronay O, Ayyar DR, et al. (2002) Demyelinating neuropathy in diabetes mellitus. Arch Neurol 59: 758-765.
- Singh K, Gupta K, Kaur S (2017) High-resolution ultrasonography of the tibial nerve in diabetic peripheral neuropathy. J Ultrason. 17: 246-252.
- Strowd R, Lycan T, Thomas A, et al. (2019) Neuromuscular ultrasound for the non-invasive assessment of breast cancer patients with peripheral neuropathy from taxanes. Neurology, 92.
- Suk JI, Walker FO, Cartwright MS (2013) Ultrasound of Peripheral Nerves. Curr Neurol Neurosci Rep 13: 328-344.
- Tan CY, Arumugam T, Omaira Razali SN, Yahya MA, et al. (2018) Nerve ultrasound can distinguish chronic inflammatory demyelinating polyneuropathy from demyelinating diabetic sensorimotor neuropathy. J Clin Neurosci 57: 198-201.
- Tawfik EA, Walker FO, Cartwright MS (2015) A pilot study of diagnostic neuromuscular ultrasound in Bell’s palsy. J Neuroimaging 25: 564-570.
- Tawfik EA, Walker FO, Cartwright MS (2015) Neuromuscular ultrasound of cranial nerves. J Clin Neurol 11: 109- 121.
- Tawfik EA (2016) Vagus nerve ultrasound in a patient with amyotrophic lateral sclerosis. Muscle Nerve 54: 978-979.
- Tawfik EA, Walker FO, Cartwright MS, El-Hilaly RA (2017) Diagnostic Ultrasound of the Vagus Nerve in Patients with Diabetes. J Neuroimaging. 27: 589-593.
- van Alfen N (2019) Nerve ultrasound in dorsal root ganglion disorders: Smaller nerves lead to bigger insights. Clin Neuro physiol 130: 550-551.
- Vinik AI, Maser RE, Mitchell BD, et al. (2003) Diabetic autonomic neuropathy. Diabetes Care 26: 1553-1579.
- Watanabe T, Ito H, Sekine A, et al. (2010) Sonographic evaluation of the peripheral nerve in diabetic patients: the relationship between nerve conduction study, echo intensity, and cross-sectional area. J Ultrasound Med 29: 697-708.
- Watkins PJ, Edmonds ME (1983) Clinical presentation of diabetic autonomic failure. In: Bannister R, ed. Autonomic Failure. Oxford: Oxford University Press 337-370.
- Yagihashi S, Yamagishi S, Wada R (2007) Pathology and Pathogenetic mechanisms of diabeti neuropathy: correlation with clinical signs and symptoms. Diabetes Res Clin Pract. 77S: S184-S189.
- Yasuda H, Terada M, Maeda K, et al. (2003) Diabetic neuropathy and nerve regeneration. Progress in Neurobiology 69: 229-285.
- Zaidman CM, Al-Lozi M, Pestronk A (2009) Peripheral nerve size in normals and patients with polyneuropathy: an ultrasound study. Muscle Nerve 40: 960-966.
Figures at a glance
Tables at a glance