Effect of Long-Term Exposure to Silver Nanoparticles on Blood Coagulation in Vivo
Received Date: June 26, 2020Accepted Date: July 21, 2020 Published Date: July 24, 2020
doi: 10.17303/jcvm.2020.6.204
Citation:Mary Gulumian (2020) Effect of Long-Term Exposure to Silver Nanoparticles on Blood Coagulation in Vivo. J Cardio Vasc Med 6: 1-9.
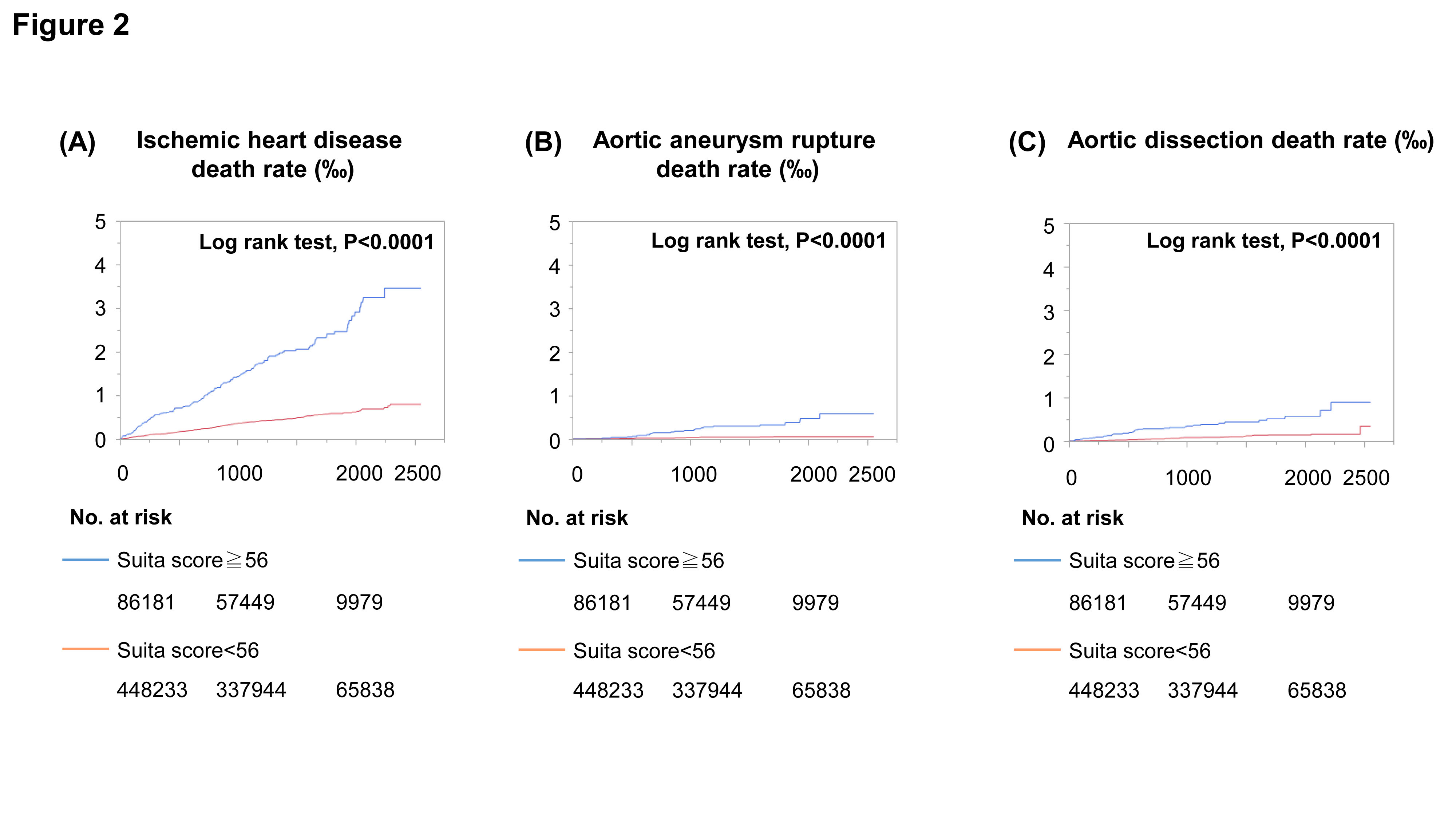
Abstract
Epidemiological studies suggest that air pollutants such as fine particulate matter (PM2.5) or ultrafine particles (UFP) (PM0.1) are responsible for cardiovascular and pulmonary adverse effects following both short-term and long-term exposures. One of the potential mechanisms may be adverse effects on blood coagulation. Because nanoparticles and ultrafine particles share a similar size range, nanoparticles may act as ultrafine particles. Silver nanoparticles (AgNPs) have been extensively used nanomaterials in consumer products and medical devices. Blood coagulation effects of long-term AgNP exposure via various routes have not been studied at all. We have analyzed our seven subacute to subchronic silver nanoparticle exposure studies conducted from 2008 to 2020 at various doses in healthy rats to investigate the effects of AgNP exposure on blood coagulation. Seven silver nanoparticle (AgNP) exposure studies including two subacute oral, one subchronic oral, one subacute inhalation, one subchronic inhalation, and two subacute intravenous injections, were analyzed. Among the seven studies, only one oral subacute study showed a significant effect on active partial thromboplastin time (aPTT) in the high dose female rats, and one subacute inhalation study showed significant effects on a PTT in all concentration groups in male rats compared to that for the control. Other studies did not show any nanoparticle exposure to relevant dose-dependent effects on blood coagulation. Therefore, the effect of subacute and subchronic exposure to AgNP on blood coagulation in the healthy rat is not clear yet.
Keywords: blood coagulation; silver nanoparticles; nanoparticles; subacute; subchronic exposure; long-term exposure
Introduction
Earlier epidemiological studies suggest that air pollutants, for instance, particulate matter (PM) have been shown to induce adverse health effects following both short-term and longterm exposures [1]. Many epidemiology studies have shown that exposure to ultrafine ambient airborne fine (PM2.5) and UFP (PM0.1) particulate matter is associated with increased mortality and morbidity, especially in elderly people with cardiovascular disease [2-3]. It could be shown that UFP could cross into the pulmonary and systemic circulations directly affecting the heart and blood vessels [4]. Controlled exposure of humans with a metabolic syndrome to concentrated UFP matter (less than 0.1 µm) caused cardiovascular effects [5]. One of the adverse effects of pulmonary exposure to UFP is changes in blood coagulation [6]. A number of investigations have therefore presented an association between exposure to UFP with inflammatory and coagulation biomarkers [7-9].
Although nanoparticles, having similar size range 1-100 nm as UFP exposure have been suspected of causing changes in blood coagulation parameters in animal exposure studies, study results are inconsistent. ZnO NPs incubated with plasma collected from healthy rats caused prolonged activated partial thromboplastin time (aPTT), where prothrombin time (PT) changed in a dose-dependent manner, but there was no size- or surface functionalization-specific pattern [10]. On the other hand, exposure to amorphous silica nanoparticles increased the activation of the coagulation cascade by adsorbing and stimulating intrinsic pathway coagulation factors and resulted in a shortening of coagulation time in aPTT and PT tests [11]. In contrast, a subacute (28-day) inhalation toxicity study of amorphous silica nanoparticles in rat resulted in no significant differences in the blood coagulation time such as prothrombin time (PT) and activated partial thromboplastin time (aPTT) between control and silica nanoparticle-exposed groups during all recovery periods [12].
Silver nanoparticles (AgNPs) are one of the most widely used nanomaterials for microbiocidal and biomedical purposes including antibacterial spraying and coating, wound dressings, and medical devices. Several consumer product databases listed AgNP containing products as the highest products among other nanoproducts [13-14]. Many of AgNP containing products have medically-related uses. Therefore, the effects of AgNP on the hemocompatibility or blood coagulation system are extremely important for medical uses of AgNPs. The effects of AgNP exposure on hemostasis in human blood are still unclear. Most studies have done using human or animal blood in vitro, or ex-vivo [15-17] or short-term in vivo studies to confirm in vitro studies [16-19]. There are only a few studies for the long-term effects of AgNPs exposure on blood coagulation in vivo. Workers, consumers, and patients might be exposed to AgNPs continuously through inhalation, oral ingestion, or intravenous administration. Therefore, we analyzed seven subacute to subchronic AgNP exposure studies to investigate blood coagulation effects caused by AgNP exposure. We analyzed seven subacute to subchronic AgNP in vivo studies which were conducted in our laboratories with various routes of administration and reported in the literature. The seven long-term silver nanoparticle (AgNP) exposure studies were three subacute oral [20-21], one subchronic oral [22] (Kim et al., 2010), one subacute inhalation [23], one subchronic inhalation [24], and one subacute intravenous injection [25-26]. These studies used various sizes of AgNPs from 10 to 60 nm and evaluated their subacute and subchronic toxicities along with hematology and platelet coagulation parameters including aPTT and PT, and blood concentration of Ag.
Materials and Methods
Nanomaterial identification, generation, and exposure routes
Nanomaterial identification including manufacturer, size distribution, suspended media, aerosol generation method, and exposure routes are described in (Table 1).
Collection of subacute and subchronic nanoparticle toxicity studies
Although we have searched NIH Pubmed databases and Google for peer-reviewed articles on blood coagulation with aPTT and PT data in in vivo subacute and subchronic animal tests, the only studies available currently are the studies that have been conducted in our lab. Other in vivo studies for inhalation, oral, and intravenous exposure studies did not analyze blood coagulation relevant parameters. Table 2 shows subacute and subchronic studies on AgNPs. All studies selected were approved by the animal care and use committees.
Quality Assessment of studies
We assessed the reliability of toxicological studies and assigned as 1 = reliable without restriction; 2 =reliable with restriction; 3 = not reliable and 4 = not assignable. Based on the data quality criteria used by the OECD WPMN dossier for nanomaterials [26] or nanomaterial hazard categorization using the OECD WPMN dossier [27]. These studies were then classified by the quality of each study as high, medium, or low (Table 3). Studies were classified as high quality if OECD WPMN rated the data with reliability class 1 or 2 and if the study was conducted in compliance with Good Laboratory Practices (GLP), based on the OECD test guideline, and/or the study was published in a peer-reviewed journal. The study was classified as medium quality if the OECD data rating was 1 or 2 but the study was not conducted in compliance with GLP, based on the OECD test guideline or not based on the OECD test guideline, but it was published in a peer-reviewed journal. The study was rated as low quality if the data were classified as OECD reliability class 4 and the study was not in compliance with GLP or the OECD test guideline and was not published in a peer-reviewed journal.
Analysis of blood coagulation results
All these studies have assessed PT and aPTT along with platelet count in the blood after subacute or subchronic exposure. The PT, aPTT, and platelet count of exposed animals were therefore analyzed for their statistical significance compared with control animals. Also, blood concentrations of nanomaterials in response to the respective dose were analyzed (Table 2).
Results
Summary and assessment of studies
Among seven peer-reviewed studies, two oral administration studies [20, 22] and one inhalation study [24] were conducted based on OECD test guidelines with the application of GLPs and rated as “1”. Other studies conducted according to the OECD test guidelines but not applied GLPs were rated as “2”.
Subacute AgNP oral administration studies
Male and female rats were exposed to AgNP (60 nm) at concentrations of 0, 30, 300, and 1000 mg/Kg body weight for 28 days under GLP according to OECD test guideline 407. Serum alkaline phosphatase and cholesterol values increased dose-dependently, indicating liver damage over 300 mg/ kg in both male and female rats. The number of platelets and the mean platelet volume did not show any dose-dependent change in male and female rats, while shortening of aPTT was noted in the high-dose (1000 mg/kg BW) female rats indicating some effects on the blood coagulation [20].
In another subacute study male and female Sprague– Dawley rats were divided into three groups: control, low (100 mg/kg body weight), and high dose (500 mg/kg body weight), and exposed orally to two different sizes (10 and 25 nm) of AgNP for 28 days. The rats were observed for 4 months of the post-exposure observation period. Platelet count decreased in the high-dose (500 mg/kg body weight) female rats (25 nm) from a two-month recovery group. Blood Ag concentrations rapidly decreased during the first month following cessation of exposure and then maintained these levels for the remainder of the 4- month recovery period. The PT increased significantly (P < 0.05) in the high-dose (25 nm) female rats when compared with the controls. However, the PT and aPTT did not show any significant changes in either the male or female rats in one and two-month recovery groups [21].
Subchronic AgNP oral administration study
A subchronic study of oral toxicity of AgNP (56 nm) in male and female F344 rats was conducted for 13 weeks (90 days) at concentrations of 0, 30, 125 and 500 mg/kg body weight under GLP according to OECD test guideline 408 [22]. Significant dose-dependent changes were found in alkaline phosphatase and cholesterol for male and female rats as seen in the subacute study [20], indicating that exposure to more than 125 mg/kg body weight of silver nanoparticles may result in slight hepatotoxicity. The number of platelets and the mean platelet volume did not show any dose-dependent change in either male or female rats. There were no dose-dependent changes in PT and aPTT in this subchronic study [22].
Subacute AgNP inhalation study
AgNPs (18.1-19.6 nm) generated from a spark generator were exposed subacutely (28-day) to male Sprague-Dawley rats at concentrations of 0, 31, 82, and 116 μg/m3 . The rats were observed for the post-exposure observation period 1 (PEO-1), 7 (PEO-), and 28-days (PEO-28). Differential blood cell counting and evaluation of inflammatory biomarkers in bronchoalveolar lavage fluid and histopathology of the lungs indicated that exposure to the high concentrations of AgNP induced inflammation at PEO-1 and at PEO-7 resolved slowly and at PEO-28 resolved completely. Mean platelet volume (MPV) decreased significantly (P < 0.01) in the moderate and high dose at PEO-7. AgNP exposure did not affect blood coagulation in terms of prothrombin time (PT) but the activated partial thrombin time (aPTT) shortened significantly (P < 0.01–0.05) at PEO-1 in all concentration groups [23].
Subchronic AgNP inhalation study
Sung et al. [24] exposed male and female Sprague-Dawley rats to AgNP (average diameter 18–19 nm) subchronically for 13 weeks at concentrations of 0, 49, 133, and 515 µg/m3 , based on the OECD test guideline 413 under GLPs. The study was conducted in a whole-body inhalation chamber. There was a clear dose-dependent increase in the silver concentration in the blood, and all tissues examined including liver, kidneys, olfactory bulb, brain, lungs, and blood for both sexes. The number of platelets and the mean platelet volume (MPV) did not show any dose-dependent change in male and female rats. There were no dose-dependent changes in PT and aPTT. The percent of erythrocyte aggregation increased in high-dose female rats [24]. This subchronic inhalation study also indicated no significant effects of AgNP exposure to blood coagulation.
Subacute AgNP intravenous injection study
Lee et al. [25-26] conducted a subacute intravenous (IV) toxicity study to investigate the effect of intravenous administration of AgNP (10 nm) at 0, 10, and 100 μg/kg body weight. The effects were examined with blood biochemistry, hematology, platelet coagulation, and nanomaterial tissue distribution. The Ag accumulated in a dose-dependent manner in the liver, spleen, kidneys, lung, brain, testis, or blood. There were no significant dose-related changes in hematology and blood biochemistry. Coagulation time in terms of the aPTT and PT did not change significantly when compared to the control group.
AgNP and AuNP mixture intravenous injection study
Lee et al. [25-26] conducted a subacute toxicity study to investigate the effect of intravenous administration of AgNP (10 nm) and AuNP (12.8 nm) and a mixture of AgNP/AuNP on blood biochemistry, hematology, platelet coagulation, and nanomaterial tissue distribution. AgNP/AuNP doses were administered at 0, 10 µg/10 μg and 100 µg/100 µg body weight. The MPV decreased in the low dose AuNP and the low dose AgNP/AuNP mixture and in the high dose AgNP/AuNP mixture after 4-weeks of recovery. Coagulation time in terms of the aPTT and PT did not change significantly compared to the control group.
Summary of observations
One oral subacute AgNP study showed shortening of aPTT was observed in the high dose (1000 mg/kg body weight) female rats [20]. Also, one subacute AgNP inhalation study showed a shortening of aPTT in all dose groups [23]. In the AgNP subchronic inhalation study, the percent of erythrocyte aggregation increased significantly in female rats in the high concentration without affecting PT and aPTT [24].
Discussion
Biomarkers for blood coagulation used in our animal studies were aPPT and PT. Activation of the intrinsic coagulation pathway is measured by shortening of aPTT and activation of the coagulation pathway, while extrinsic pathway is measured by PT. This coagulation pathway is activated when the tissue factor is released after tissue damage to induce a downstream coagulation cascade. In this paper, we analyzed in vivo toxicity data on AgNPs from subacute to subchronic studies using various routes obtained from selected quality publications and determined whether AgNP subacute and subchronic exposure can influence blood coagulative process. In the studies analyzed, Ag ions or AgNPs or secondary AgNPs generated from Ag ions reacting with biogenic macromolecules might be translocated into the blood and to other tissues [23]. Our analysis indicated that there was no consistent, dose-dependent and distinct effect on blood coagulation from subacute and subchronic exposures of AgNP administered via different routes including oral, inhalation, and intravenous administration. There was also no consistent effects on biomarkers for blood coagulation used in the studies (PT, aPTT, and platelet count). Shortening of aPTT (relevant to blood coagulation) was observed in one oral subacute AgNP in high dose (1000 mg/kg body weight) female rats [20] and in one inhalation study (31, 82, and 116 μg/m3 ) [23]. In this subacute oral study, the blood concentration of Ag was very high showing 0.7 µg/g of blood in females. However, no changes in aPTT were observed in male rats with blood Ag (0.8 µg/g) higher than female rats. In the AgNP subchronic inhalation study [24], erythrocyte aggregation increased significantly in the high concentration in female rats without affecting PT and aPTT. The blood concentration in the high dose female rats was 0.0069 µg/g, 100-fold less than observed in the subacute oral study. In a subacute intravenous injection study [25-26], AgNP alone or mixture with gold nanoparticle intravenous injection caused decreases in MPV without affecting PT and aPTT, but the effects were not dose-dependent.
It is important to note that the composition of AgNP aerosol may influence blood coagulation. Jo et al [23] used AgNPs generated from a spark generator without a supply of inert gas. The AgNP content was 70% in weight with other impurities such as Si (16.2%) and S (9.1%) detected with TEM-EDX (energy dispersive X-ray) analysis. In contrast, a subchronic inhalation study by Sung et al [24] used AgNPs generated from an evaporation/condensation generator. The AgNP content was higher than 99% without significant impurities. This study showed the increased percent of erythrocyte aggregation in the high concentration females only. Therefore, some of the impurities in the AgNPs generated from a spark may contribute to the shortening of aPTT in this study. Also, the study by Jo et al [23] suggested a role for secondary insoluble AgNP generated by Ag ions reacting with biomolecules in lung clearance and translocation to other tissues. Their study indicated that the initial fast clearance of Ag from the lung was dissolution dependent and the slow clearance was mainly the removal of secondary AgNP from the lungs. It is not clear whether in the blood after inhalation exposure were AgNPs translocated to the blood from the lung crossing the airblood barrier or secondary AgNP formed from Ag ions. The roles of secondary AgNPs or Ag ion in blood coagulation are not known currently. A recent study by Bian et al [19] suggested that amount of Ag ion released from AgNP was negligible and Ag ion did not induce phosphatidylserine exposure although the dissolution may differ in various physicochemical states.
Inhalation and intravenous injection can both introduce nanoparticles directly into the blood stream. These nanoparticles can contact blood components including platelets and coagulation factors which have the potential for stimulating platelet aggregation and thrombosis or blood clotting disorders leading to bleeding [29]. In our previous study, blood concentrations of Ag were 1.64 and 15.97 ng/g of blood after 28-day caudal vein injection of AgNP at concentrations of 10 μg/kg and 100 μg/kg body weight, respectively, resulted in no effect on aPTT and PT [26]. Thus, a high concentration of AgNPs in the bloodstream did not affect blood coagulation. Furthermore, 28-day co-injection of AgNP and AuNP (gold nanoparticles) at concentrations of AgNP/AuNP 10/10 μg/kg and 100/100 μg/kg body weight did not affect at all blood coagulation [26]. These studies clearly indicated that the presence of AgNP in the blood may not be a prerequisite for the blood coagulation. Some other factors relevant to inflammation would be required to induce blood coagulation as seen in the subacute oral dosing study [20] and subacute inhalation study [22] in which AgNPs were contaminated by other chemicals.
It is therefore not possible to draw a clear conclusion on the effects of AgNPs on the blood coagulation system. Few studies on in vivo combining with in vivo or ex-vivo studies of AgNP effects on blood coagulation have been reported. For example, AgNP induced platelet aggregation and procoagulant activity in freshly isolated human platelets in vitro. Furthermore, AgNP 0.05-0.1 mg/kg bw intravenous injection or 5-10 mg/kg bw intratracheal instillation enhanced venous thrombus formation, platelet aggregation, and phosphatidylserine externalization ex vivo [18]. AgNP promoted phosphatidylserine exposure and microvesicle generation in freshly isolated human RBCs mainly by reactive oxygen species generation and intracellular calcium increase. Moreover, this AgNP-induced procoagulant activity of RBC was observed at non-hemolytic concentrations [19]. In contrast, several researchers reported that AgNPs inhibited platelet activation, adhesion, aggregation, and thrombosis [30-33]. The lack of effect in our long-term studies might be attributed to the use of healthy animals, the time points evaluated, inflammatory response, and adopted response due to prolonged exposure.
Conclusions
We have reviewed silver nanoparticle subacute to subchronic exposure studies in healthy rats to investigate the effects of nanoparticle exposure on blood coagulation. There was no consistent and distinct effect on blood coagulation from subacute and subchronic exposures of AgNPs administered via different routes including oral, inhalation, and intravenous administration.
Availability of supporting data
All data and materials are included in the manuscript, tables, figures, and supplements
Competing interests
The authors declare that they have no competing interests.
Funding
This study was supported by the Global Top Environment Technology Development project (No. 2018001860004) funded by the Ministry of Environment and EU H2020, NanoSolveIT Project.
Authors’ contributions
GM, review data and manuscript preparation; BK, review data and manuscript preparation; JSC, review data; CHK, review data; IJY, review data, and manuscript preparation. All authors read and approved the final manuscript.
- (2005) WHO. WHO Air quality guidelines for particulate matter, ozone, nitrogen dioxide, and sulfur dioxide, Global update 2005: Summary of risk assessment, WHO, Geneva.
- EPA US (2009) Integrated science assessment for particulate matter (final report). Washington, DC, EPA/600/R-08/139F
- Pope CA, Burnett RT, Thurston GD, Thun MJ, Calle EE, Krewski D, Godleski JJ (2004) Cardiovascular mortality and long-term exposure to particulate air pollution: epidemiological evidence of general pathophysiological pathways of disease. Circulation 109: 71–77.
- Nelin TD, Joseph AM, Gorr MW Loren E (2012) Direct and Indirect Effects of PM on the Cardiovascular System Toxicol Lett 208: 293–299.
- Devlin RB, Smith CB, Schmitt MT, Rappold AG, Hinderliter A, et al. (2014) Controlled exposure of humans with metabolic syndrome to concentrated ultrafine ambient particulate matter causes cardiovascular effects. Toxicol. Sci.140: 61–72.
- Lippi G, Favaloro, EJ, Franchini M, Guidi GC (2008) Air pollution and coagulation testing: a new source of biological variability? Thromb. Res. 123: 50-54.
- Rückerl R, Phipps RP, Schneider A, Frampton M, Cyrys J, Oberdörster G (2008) Ultrafine particles and platelet activation in patients with coronary heart disease – results from a prospective panel study. Particle and Fibre Toxicology 4: 1.
- Lane KJ, Levy JI, Scammell MK, Peters JL, Patton AP, Reisner E, Lowe L, et al. (2016) Association of modeled long-term personal exposure to ultrafine particles with inflammatory and coagulation biomarkers. Environment International 92–93: 173- 182.
- Liu C, Jing C, Qiao L, Wang H, Xu W, Li H, Zhao Z, Chen R, Kan H (2017) The Acute Effects of Fine Particulate Matter Constituents on Blood Inflammation and Coagulation, Environ. Sci. Technol 51: 8128-8137.
- Yang JY, Bae J, Jung A, Park S, Chung S, Seok J, Roh H, Han Y, et al. (2017) Surface functionalization-specific binding of coagulation factors by zinc oxide nanoparticles delays coagulation time and reduces thrombin generation potential in vitro. PLoS One 12: e0181634.
- Gryshchuk V, Galaga N (2016) Silica Nanoparticles Effects on Blood Coagulation Proteins and Platelets. Biomedical Research International.
- Shin JH, Jeon K, Kim JK, Kim Y, Jo MS, Lee JS, Baek JE, et al. (2017) Subacute inhalation toxicity study of synthetic amorphous silica nanoparticles in Sprague-Dawley rats. Inhal Toxicol 29: 567-576.
- Woodrow Wilson International Center for Scholars (2015). The project on emerging nanotechnologies. Available at: https://www.nanotechproject.org/inventories
- The Nanodatabase (2020), https://nanodb.dk/ accessed at 2/20/2020.
- Laloy J, Minet V, Alpan L, Mullier F, Beken S, et al. (2014) Impact of Silver Nanoparticles on Haemolysis, Platelet Function, and Coagulation. Nanobiomedicine.
- Weber M, Steinle H, Golombek S, Hann L, Schlensak C, et al. (2018) Blood-Contacting Biomaterials: In Vitro Evaluation of the Hemocompatibility. Front BioengBiotechnol 6.
- Simak J, De Paoli S (2017) The effects of nanomaterials on blood coagulation in hemostasis and thrombosis. Wiley Interdiscip Rev Nanomedicine Nanobiotechnology 9: e1448.
- Jun EA, Lim KM, Kim K, Bae ON, Noh JY, et al. (2011) Silver nanoparticles enhance thrombus formation through increased platelet aggregation and procoagulant activity. Nanotoxicology 5: 157–67.
- Bian Y, Kim K, Ngo T, Kim I, Bae ON, et al. (2019) Silver nanoparticles promote the procoagulant activity of red blood cells: a potential risk of thrombosis in a susceptible population. Particle and Fibre Toxicology 16: 9.
- Kim YS, Kim JS, Cho HS, Rha DS, Kim JM, Park JD, et al. (2008) Twenty-eight-day oral toxicity, genotoxicity, and gender-related tissue distribution of silver nanoparticles in Sprague-Dawley Rats, Inhalation Toxicology, 20: 575-583.
- Lee JH, Kim YS, Song KS, Ryu HR, Sung JH, et al. (2013) Biopersistence of silver nanoparticles in tissues from Sprague-Dawley rats, Particle and Fibre Toxicology 10:36.
- Kim YS, Song MY, Park JD, Song KS, Ryu HR, et al. (2010) Subchronic oral toxicity of silver nanoparticles. Part Fibre Toxicol. 7:20
- Jo MS, Kim JK, Kim Y, Kim HP, Kim HS, et al. (2020) Mode of silver clearance following 28-day inhalation exposure to silver nanoparticles determined from lung burden assessment including post-exposure observation periods, Archive of Toxicology 94: 773-784.
- Sung JH, Ji JH, Park JD, Yoon JU, Kim DS, et al. (2009) Subchronic inhalation toxicity of silver nanoparticles. Toxicol Sci. 108: 452-461
- Lee JH, Sung JH, Ryu HR, Song KS, Song NW, Park HM, (2018) Tissue distribution of gold and silver after subacute intravenous injection of co-administered gold and silver nanoparticles of similar sizes. Arch Toxicol 92: 1393-1405.
- Lee JH, Gulumian M, Faustman EM, Workman T, Jeon K, et al. (2018) Blood Biochemical and Hematological Study after Subacute Intravenous Injection of Gold and Silver Nanoparticles and Co administered Gold and Silver Nanoparticles of Similar Sizes. Biomed Research International, Article ID 8460910.
- OECD (2018) Testing Programme of Manufactured Nanomaterials, OECD, Paris.
- Lee N, Lim CH, Kim T, Son EK, Chung GS, Rho CJ, et al. (2017) Systemic evidence review for developing WHO guidelines on protecting workers from potential risks of manufactured nanomaterials: Which hazard category should specific nanomaterials or groups of nanomaterials be assigned to and how? WHO/FWC/IHE/17.4, WHO, Geneva.
- Tomaszewski KA, Radomski MW, Santos-Martinez MJ (2015) Nano diagnostics, nanopharmacology and nanotoxicology of platelet-vessel wall interactions. Nanomedicine (Lond) 10: 1451-75.
- Ragaseema VM, Unnikrishnan S, Krishnan VK, Krishnan LK. (2012) The antithrombotic and antimicrobial properties of PEG-protected silver nanoparticles coated surfaces. Biomaterials 33: 3083-3092.
- Shrivastava S, Bera T, Singh SK, Singh G, Ramachandra rao P, Dash D (2009) Characterization of Antiplatelet Properties of Silver Nanoparticles. ACS Nano 3: 1357–1364.
- Huang H, Lai W, Cui M, Liang L, Lin Y, et al. (2016) An evaluation of blood compatibility of silver nanoparticles. Scientific Reports 6: 25515.
- Martínez-Gutierrez F, Thi EP, Silverman JM, de Oliveira CC, et al. (2012) Antibacterial activity, inflammatory response, coagulation, and cytotoxicity effects of silver nanoparticles. Nanomedicine. 8: 328-336.
Tables at a glance